Photovoltaic Powered Hydrogen Plant
DESIGN PROJECT
This final design project for ENGN 0720: Thermodynamics integrated the knowledge learned in the course on thermodynamic cycles to design a functional hydrogen production plant. In particular, an analysis was carried out on turbines, compressors, heat exchangers, pumps, chemical equilibria, and separation processes all in their no-ideal states to rigorously model the output and profitability of a hydrogen plant under the design restrictions given. The open-ended nature of the project, however, mirrored a real-life scenario and allowed our team to come up with creatively engineered solutions.
PROBLEM STATEMENT
Design and optimize a thermodynamically accurate process to produce and sell hydrogen and potentially steam. Use the net present value method to calculate profitability.
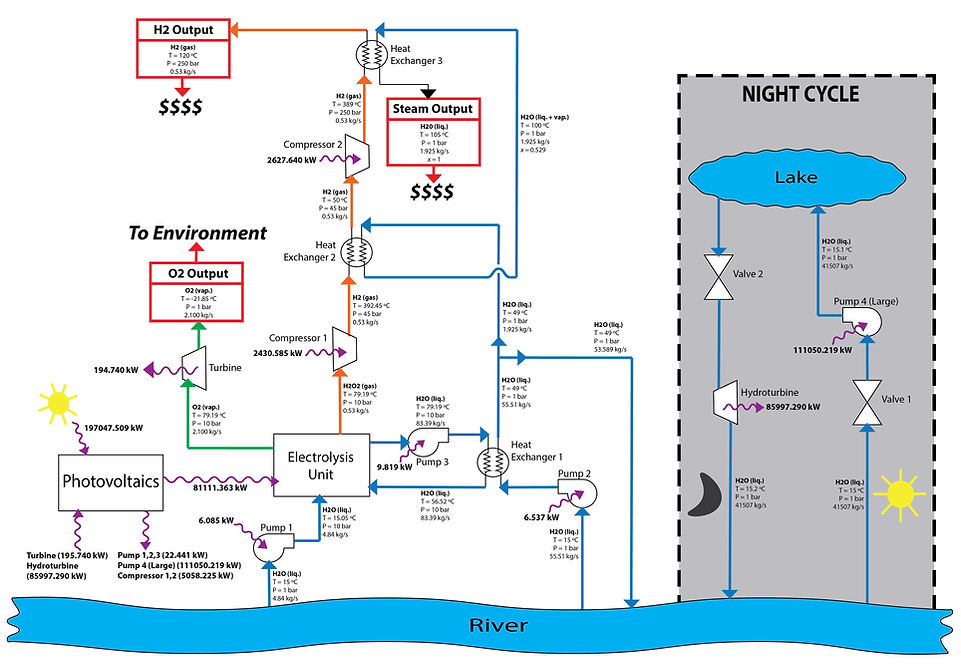.jpg)
Since the thermodynamic processes at play in the production of hydrogen from photovoltaic electrolysis are non-trivial, we first focused on creating a thermodynamically sound process that met the design restrictions. To do this, we determined the power that would have to be supplied by the photovoltaic cells to power the electrolysis unit. We then set the electrolysis temperature to ensure the hydrogen output would only be 4% contaminated by using partial pressures. A series of compressors and heat exchangers were then added in order to output the hydrogen at the required pressure and temperature. Careful consideration was given to the maximum working temperature of the compressors.
From this stage, we worked on maximizing the profitability of the hydrogen production plant. In order to maximize sales and decrease equipment costs, we optimized the sale of steam from the post-electrolysis heat exchangers. This was accomplished by decreasing the temperature of the output stream to 100 degrees Celsius. We created a Matlab script that would optimize the inlet and outlet temperatures (where applicable) of our heat exchangers, which, once applied, raised our overall NPV by reducing the cost of our heat exchangers. After these iterations, we decided to implement a night cycle, which would almost double our NPV (the $11 million infrastructure costs are easily offset by the increase in production profits). The night cycle consists of a solar- powered pump that transports river water to an elevated lake during the day; this water is then let run (valve-controlled) through a hydro turbine at night, which produces enough power to run the entire cycle, so that we are able to continuously produce and sell steam and H2 over a 24 hour period. The next change we made was the addition of a heat exchanger before the sale of H2, which will, in turn, produce more steam for sale. Each added heat-exchanger runs with a counter current system, which reduces the overall area of the exchangers and therefore cost of the plant.
After finalizing the physical cycle, we took advantage of different depreciation rates in calculating the Net Present Value. We ended up using a double depreciation model for year one, a sum of years model for years 2-5, and a straight line model for years 6-10. These changes netted us an estimated 617 million NPV after ten years.